The Battery Runtime Calculator is an indispensable tool for anyone using batteries for power supply, be it in RVs, boats, off-grid systems, or even in everyday electronics. This calculator simplifies the process of determining how long a battery will last under specific conditions. It features inputs for battery capacity, voltage, type, state of charge, depth of discharge limit, inverter usage, and total output load. There’s a clear result display and a reset button for convenience. We have recently added some Advanced Features. Try it! p.s. we also just published our Battery Runtime Toolkit – with some extra Bonus content. Check it out below!
Table of Contents
Battery Runtime is not just about knowing the duration; it’s about planning, efficiency, and making the most out of your battery’s potential. Understanding and utilizing Battery Runtime Calculator can significantly enhance your battery usage knowledge, and prevent you from having worries about making it to your goal in a car, or charging your solar batteries. Check your Solar Panel Size using this calculator!
- Solar Panel Size Calculator
- Check Maximum Power Output with Solar Panel Output Calculator
- Solar Watts to Amps Converter
- While here, try our How Long Ago Calculator
- Sent a package? Calculate its USPS shipping times!
How to Use the Battery Runtime Calculator
Below is a detailed explanation of how to use the Battery Runtime Calculator. The Battery Runtime Calculator helps you estimate how long your battery will power your devices under various conditions. Follow the steps below to get the most accurate runtime estimate.
Enter Your Basic Inputs
- Battery Capacity (Ah):
- Input the total amp-hour rating of your battery (e.g., 100 Ah).
- This is often printed on the battery or provided in the manufacturer’s documentation.
- Battery Voltage (V):
- Enter the nominal voltage of your battery system (e.g., 12 V, 24 V, etc.).
Set the State of Charge and Depth of Discharge
- State of Charge (%):
- Use the slider to indicate how fully charged your battery is at the start. For instance,
80%
means the battery is 80% charged.- Depth of Discharge Limit (%):
- Use this slider to define how much of your battery capacity you plan to use before stopping. For example, if you don’t want to discharge below 50%, set it to
50%
.These two sliders combine to calculate the effective portion of your battery capacity that’s usable.
Indicate Inverter Usage
- Using Inverter?
- If your devices run on AC power, you likely use an inverter. Select Yes to reveal the Inverter Efficiency slider.
- Enter the inverter’s efficiency as a percentage (e.g.,
90%
). The calculator will adjust the runtime accordingly to account for inverter losses.Provide Your Output Load
- Total Output Load (W):
- Enter the wattage of the devices you’re powering. If you have multiple devices, add their wattages together. For example, if you have a 300 W TV and a 200 W fan, your total load is 500 W.
(Optional) Enable Advanced Options
Click Show Advanced Options if you want a more realistic estimate. You can specify:
- Battery Age (years):
- Older batteries have reduced capacity. The calculator applies a 3% annual capacity loss, never reducing the battery below 50% of its original capacity.
- Ambient Temperature (°C):
- Batteries perform best around 25°C. For every degree away from 25, the calculator reduces capacity by 1%, down to a minimum of 70%.
- Load Variation (%):
- If your load fluctuates (e.g., lights or fans turning on/off), enter a percentage that represents typical variation.
(Optional) Apply Peukert’s Law
- Apply Peukert’s Law Correction:
- If your battery is discharged at higher-than-rated currents, Peukert’s law helps account for additional losses. By default, the calculator uses a 20-hour rating and a Peukert exponent of 1.15—common for lead-acid batteries.
- Check this box if you want to refine your runtime estimate for higher discharge currents.
Calculate Your Runtime
- Click “Calculate”:
- The calculator shows a brief “Calculating…” spinner.
- After a few seconds, you’ll see:
- Estimated Runtime in hours, minutes, and seconds.
- Interpretation text explaining which factors (inverter, advanced options, Peukert’s law) were used.
- Review the Results:
- The runtime is an approximation. Real-world performance may differ due to battery health, temperature fluctuations, and other factors.
Get your Battery Runtime Toolkit
Below the results, you’ll see a “Get the Battery Runtime Toolkit!” button. This leads to an in-depth guide on battery sizing, usage, and best practices. If you need more advanced strategies or want to learn how to extend your battery life, click the button to visit the sales page and explore the comprehensive toolkit.
Reset if Needed
- Click “Reset” to clear all fields and start over. This is useful if you want to perform multiple calculations with different values.
Tip:
For maximum accuracy, use up-to-date battery capacity info and measure your load precisely with a wattmeter or similar tool. The advanced options are optional but can significantly improve the estimate for older batteries, extreme temperatures, or varying loads.
Detailed Overview of Battery Types
Lead-Acid Batteries
Lead-acid batteries are one of the oldest and most widely used rechargeable battery technologies. They are known for their affordability, ability to handle high surge currents, and relatively simple charging requirements.
Subtypes of Lead-Acid Batteries:
- Flooded Lead-Acid (FLA): Requires periodic maintenance (topping up with distilled water). Common in deep-cycle applications such as off-grid solar systems and backup power storage.
- Absorbent Glass Mat (AGM): A sealed version with no maintenance required. Used in UPS systems, RVs, and marine applications.
- Gel Batteries: A type of sealed lead-acid battery where the electrolyte is in gel form. Offers better deep-cycle performance but is sensitive to charging rates.
Pros & Cons of Lead-Acid Batteries:
✔ Pros:
- Cost-effective and widely available
- Can deliver high surge currents, making them suitable for engine starting
- Can be used in deep-cycle applications
✖ Cons:
- Heavy and bulky compared to newer battery technologies
- Shorter lifespan (typically 3-5 years)
- Flooded lead-acid types require maintenance
Best For: Stationary solar systems, backup power, automotive applications, and uninterruptible power supplies (UPS).
Lithium-Ion Batteries
Lithium-ion (Li-ion) batteries have become the dominant choice in modern electronics, electric vehicles (EVs), and high-performance energy storage systems. They offer superior energy density, longer lifespan, and lighter weight compared to lead-acid batteries.
Subtypes of Lithium-Ion Batteries:
- Lithium Cobalt Oxide (LiCoO₂) – High energy density, common in laptops and smartphones.
- Lithium Iron Phosphate (LiFePO₄) – Known for safety and long cycle life, used in solar storage and EVs.
- Lithium Manganese Oxide (LiMn₂O₄) – Offers thermal stability, used in power tools and medical devices.
Pros & Cons of Lithium-Ion Batteries:
✔ Pros:
- High energy density (lightweight for the same capacity)
- Longer lifespan (up to 10+ years with proper care)
- Faster charging times
- No maintenance required
✖ Cons:
- Higher upfront cost
- Requires a Battery Management System (BMS) to prevent overcharging/discharging
- Some chemistries (e.g., LiCoO₂) are prone to overheating if not managed properly
Best For: Applications where weight, efficiency, and longevity are important, such as electric vehicles (EVs), portable electronics, and advanced solar energy storage.
Nickel-Metal Hydride (NiMH) Batteries
NiMH batteries are an improvement over older nickel-cadmium (NiCd) batteries, offering better energy density and environmental friendliness. They are commonly used in power tools, hybrid vehicles, and rechargeable AA/AAA batteries.
Pros & Cons of NiMH Batteries:
✔ Pros:
- Moderate lifespan and rechargeability
- Safer than lithium-ion batteries in some cases (lower risk of thermal runaway)
- No toxic heavy metals (unlike NiCd, which contains cadmium)
✖ Cons:
- Lower energy density compared to lithium-ion
- Higher self-discharge rate when not in use
- Less efficient compared to Li-ion in high-drain applications
Best For: Devices where safety is a higher priority than maximum energy storage, such as power tools, hybrid vehicle battery packs, and consumer electronics.
Choosing the Right Battery for Your Needs
When selecting a battery, consider the following factors:
✅ Application Needs – Are you using it for backup power, transportation, or electronics?
✅ Energy Density – Do you need a lightweight battery with high storage capacity?
✅ Lifespan & Cycle Life – How long should the battery last before needing replacement?
✅ Cost vs. Performance – Are you prioritizing affordability over efficiency?
Quick Recommendations:
- Lead-Acid: Best for stationary solar systems and backup power where weight isn’t a concern.
- Lithium-Ion: Ideal for high-efficiency applications such as EVs, solar storage, and portable electronics.
- NiMH: Suitable for power tools and hybrid vehicles, where safety and moderate lifespan matter more than energy density.
By understanding battery chemistries and their strengths and weaknesses, you can make an informed decision that maximizes efficiency, cost-effectiveness, and reliability.
Battery Basics Explained – Types of Batteries
(This section was already expanded in detail earlier, covering Lead-Acid, Lithium-Ion, and NiMH batteries.)
Key Terms Explained
Understanding battery performance requires familiarity with key terms commonly used in specifications and technical discussions.
Voltage (V)
Voltage represents the electrical potential difference of a battery. It determines the force with which electricity flows. Common battery voltages include:
- 1.2V – Typical for NiMH rechargeable AA/AAA batteries
- 3.7V – Standard for lithium-ion single-cell batteries (phones, laptops)
- 12V – Automotive and small solar applications
- 24V, 48V, 72V – Used in larger solar, electric vehicle, and industrial systems
Why It Matters:
Voltage must match the requirements of the device or system. For example, a 12V lead-acid battery bank is common for off-grid solar systems, while electric vehicles often use 48V or higher.
Capacity (Ah – Ampere-hour)
Capacity represents the amount of charge a battery can store and deliver over time.
- A 100Ah battery can theoretically provide 100 amps for 1 hour or 10 amps for 10 hours before being fully discharged (at an ideal, steady rate).
- Capacity is often used to estimate how long a battery will last under a given load.
Example:
A 12V 100Ah battery provides 1,200 watt-hours (Wh) of energy:
Power (W – Watts)
Power is the rate at which energy is consumed or delivered. It’s calculated using:
For example, a 12V battery delivering 10A produces 120W of power.
Why It Matters:
- High-power applications (e.g., electric vehicles, inverters) require batteries with high power output.
- If a battery is undersized for the load, it will discharge quickly and may overheat.
State of Charge (SoC) & Depth of Discharge (DoD)
- State of Charge (SoC): The percentage of charge left in a battery. A 100% SoC means the battery is fully charged.
- Depth of Discharge (DoD): The percentage of the battery’s capacity that has been used. A 50% DoD means the battery has used half of its charge.
Why It Matters:
- Lead-acid batteries should not be discharged below 50% DoD to maximize lifespan.
- Lithium-ion batteries can typically handle 80-90% DoD without significant lifespan reduction.
Battery Chemistry and Its Impact on Performance
Battery chemistry influences energy density, charging speed, lifespan, and safety.
Energy Density
Energy density refers to how much energy a battery can store relative to its size and weight.
Higher energy density means lighter batteries for the same capacity, making lithium-ion the best choice for portable applications.
Charging Speed
Charging time varies by chemistry:
Lithium-ion batteries charge faster, making them ideal for quick-turnaround applications.
Lifespan and Cycle Life
Battery lifespan is measured in cycles – how many charge-discharge cycles a battery can handle before capacity degrades significantly.
Battery Safety Tips
To prevent accidents and prolong battery life:
✔ Avoid overcharging: Overcharging can lead to overheating and reduced lifespan. Use a Battery Management System (BMS) for lithium-ion batteries.
✔ Prevent deep discharges: Repeatedly draining a battery to 0% reduces its lifespan, especially for lead-acid.
✔ Use proper charging equipment: Incorrect chargers can overheat or damage batteries.
✔ Store batteries properly: Keep them in a cool, dry place. Avoid high temperatures, which degrade battery chemistry.
✔ Avoid physical damage: Puncturing or crushing batteries (especially lithium-ion) can cause fires.
Battery Lifespan Management – How to Extend Battery Life:
- Use a compatible charger – Overvoltage or undervoltage damages cells.
- Avoid extreme temperatures – Store between 10°C and 30°C (50°F to 86°F).
- Limit charge cycles – Don’t frequently deep discharge lead-acid batteries.
- Proper storage – If storing batteries long-term, keep them at 50% charge to avoid degradation.
Emerging Battery Technologies
Battery technology is evolving, with new chemistries and designs improving efficiency, safety, and sustainability.
- Solid-State Batteries: No liquid electrolyte, safer, with higher energy density. Expected to replace lithium-ion in EVs.
- Sodium-Ion Batteries: Cheaper and more environmentally friendly than lithium-ion, with potential for mass energy storage.
- Graphene Batteries: High conductivity, ultra-fast charging, and long lifespan (still in research phase).
Common Battery Myths Debunked
🚫 “You should always fully discharge a battery before recharging.”
❌ False – Modern batteries (especially lithium-ion) prefer shallow discharge cycles.
🚫 “Cold temperatures kill batteries instantly.”
❌ Partly true – Cold reduces efficiency, but batteries recover once warmed up.
🚫 “Bigger batteries always last longer.”
❌ Not necessarily – Lifespan depends on usage, depth of discharge, and chemistry.
Conclusion:
By understanding battery types, key terms, chemistry impacts, and proper care, you can maximize performance, lifespan, and safety across various applications.
Diagram above shows: Voltage vs. Capacity in Different Battery Types
This bar chart shows how different battery chemistries vary in terms of capacity (Ah). Lithium-ion typically offers the highest capacity per unit size, followed by lead-acid and NiMH.
Flowchart: Choosing the Right Battery Type
This flowchart helps you determine the best battery type based on your needs. If high power and portability are priorities, Lithium-Ion is the best choice. If deep-cycle capability is more important, Lead-Acid is recommended. For moderate power and safety, NiMH can be a good option.
Next, we’ll show a table comparing battery lifespan across different Depths of Discharge (DoD).
Battery Lifespan vs. Depth of Discharge (DoD)
Above is a table comparing battery lifespan across different Depths of Discharge (DoD). You can now review it for insights on how different battery types perform over time based on their discharge limits.
Charging Time Comparison of Battery Types
Charging time is often a key factor when choosing a battery.
As shown in the chart, Lead-Acid batteries are the slowest to charge, often taking around 8-12 hours to reach 80% capacity. NiMH batteries are faster, typically taking 2-4 hours, making them a good middle-ground solution. However, Lithium-Ion batteries charge the fastest – some can reach 80% capacity in just 1-2 hours – perfect for applications where quick turnaround is essential, like electric vehicles or portable electronics.
Battery Safety Tips
Proper battery care ensures longevity, efficiency, and most importantly, safety. Here’s a quick guide to handling batteries safely:
- Avoid Overcharging – Always use a compatible charger to prevent overheating or damage.
- Prevent Deep Discharges – Discharging too deeply shortens battery lifespan, especially for lead-acid types.
- Use the Right Charger – Incorrect voltage or current can harm your battery or even cause hazards.
- Store Properly – A cool, dry place is best for storing batteries long-term.
- Avoid Physical Damage – Damaged batteries can leak chemicals or catch fire, particularly lithium-ion batteries.
Battery Lifespan vs. Temperature
Temperature plays a critical role in how long your battery lasts. This graph illustrates how different battery types perform under varying temperatures:
- Lead-Acid batteries perform well at moderate temperatures (around 20°C), but their lifespan drops sharply in both extreme cold (-20°C) and high heat (40°C).
- Lithium-Ion batteries maintain good performance in a wider range but are significantly impacted by extreme cold, where their lifespan can drop by up to 70%.
- NiMH batteries offer balanced performance across the spectrum, though not as efficient as lithium-ion in moderate conditions, making them reliable in fluctuating environments.
Takeaway:
To maximize your battery’s lifespan, keep it within the recommended temperature range. Extreme heat accelerates chemical wear, while extreme cold slows down chemical reactions, reducing efficiency.
Lifespan of Emerging Battery Technologies
Emerging Battery Technologies
As technology evolves, new battery types are emerging with incredible potential:
- Solid-State Batteries: Offering higher energy density (up to 400 Wh/kg) and around 10,000 charge cycles, these batteries are set to revolutionize EVs due to their safety and compact size.
- Sodium-Ion Batteries: A cost-effective alternative to lithium, with a decent energy density of 150 Wh/kg and around 5,000 cycles, making them ideal for grid storage.
- Graphene Batteries: The future of high-performance energy storage, graphene boasts an impressive 600 Wh/kg energy density and up to 15,000 cycles, although still in the research phase.
- Lithium-Ion Batteries (Current Benchmark): With 250 Wh/kg and about 5,000 cycles, they remain the gold standard for now, especially in consumer electronics and EVs.
Next, let’s explore a battery cost comparison to help you balance performance with affordability!
Battery Cost Comparison (USD per kWh)
Shown above is Battery Cost Comparison (USD per kWh). Cost is often the deciding factor when choosing a battery. Here’s how different types compare:
- Lead-Acid batteries are the most affordable (~$150/kWh), making them a popular choice for budget-conscious applications like backup power.
- NiMH sits in the mid-range at ~$200/kWh, offering decent performance for its price.
- Lithium-Ion batteries cost more (~$400/kWh) due to their higher energy density and longer lifespan.
- Sodium-Ion presents a promising future with lower costs (~$300/kWh), potentially challenging lithium-ion.
- Solid-State batteries, while offering superior performance, are currently the most expensive at ~$800/kWh due to complex manufacturing processes—but prices are expected to drop as the technology matures.
Tip for readers: If you’re looking for the best balance of cost and performance today, lithium-ion is your best bet, but keep an eye on sodium-ion and solid-state advancements!
Formula Behind the Battery Runtime Calculator
The formula behind the Battery Runtime Calculator is grounded in basic electrical principles. The key formula is:

This calculation considers:
- Battery Capacity (Ah): The total charge the battery can hold.
- State of Charge (SoC): The current charge level of the battery as a percentage.
- Depth of Discharge (DoD): The percentage of the battery that has been or can be discharged relative to its total capacity.
- Total Output Load (W): The total power demand from the connected devices.
- Battery Voltage (V): The voltage rating of the battery.
Step-by-Step Calculation Guide
Example Scenario: A 12V 100Ah Lead-Acid Battery
- Enter Battery Capacity: 100Ah
- Enter Battery Voltage: 12V
- Select Battery Type: Lead-acid
- Enter State of Charge: 100% (Fully charged)
- Enter Depth of Discharge Limit: 50% (Recommended for lead-acid)
- Inverter Usage: No
- Enter Total Output Load: 120W
Calculation:
The runtime is calculated as:

So, the battery will last approximately 5 hours under these conditions.
Definition and Background of Battery Runtime Calculator
Battery runtime refers to the duration a battery can power devices before needing a recharge. This concept is crucial in scenarios where consistent power supply is essential, such as in emergency systems, renewable energy storage, and mobile applications.
The runtime depends on several factors:
- Battery Capacity: Measured in amp-hours (Ah), it indicates how much charge the battery can hold.
- Voltage: This determines the energy per unit charge the battery can deliver.
- Battery Type: Different types have varying efficiencies and discharge characteristics.
- State of Charge (SoC): Indicates how full the battery is.
- Depth of Discharge (DoD): Represents how much of the battery’s capacity is utilized.
- Load: The power demand from connected devices affects how quickly the battery drains.
In real-world applications, battery runtime calculations are essential for designing backup power systems, planning energy usage in off-grid setups, and ensuring the longevity of battery-powered devices.
Table of Example Calculations for Battery Runtime
Battery Type | Capacity (Ah) | Voltage (V) | SoC (%) | DoD Limit (%) | Load (W) | Runtime (hours) |
---|---|---|---|---|---|---|
Lead-acid | 100 | 12 | 100 | 50 | 120 | 5 |
Lithium | 100 | 12 | 80 | 100 | 200 | 4.8 |
Lead-acid | 200 | 24 | 60 | 50 | 300 | 4 |
Lithium | 50 | 48 | 100 | 100 | 500 | 0.96 |
Explanation of the Table
This table showcases various scenarios using different battery types, capacities, states of charge, depth of discharge limits, and loads. It helps users understand how these factors interact to determine the runtime of a battery. For example, a 100Ah lead-acid battery at 12V with a 100% state of charge and a 50% DoD limit can run a 120W load for 5 hours.
Glossary for Battery Runtime Calculator
- Ampere-hour (Ah): A unit of electric charge.
- Voltage (V): Electric potential difference or electromotive force.
- State of Charge (SoC): The current level of charge in a battery as a percentage of its capacity.
- Depth of Discharge (DoD): The percentage of the battery that has been discharged relative to its total capacity.
- Load (W): The power demand from connected devices.
Thoroughly Detailed Step-by-Step Guide to Using the Battery Runtime Calculator
- Input Battery Capacity:
Locate the field labeled “Battery Capacity (Ah)”. Enter the capacity of your battery in ampere-hours (Ah). This is a measure of how much charge the battery can hold.
2. Input Battery Voltage:
Find the field labeled “Battery Voltage (V)”. Enter the voltage of your battery in volts (V). This indicates the electrical potential difference of the battery.
3. Input State of Charge:
Look for the field labeled “State of Charge (%)”. Enter the current state of charge of your battery as a percentage (%). This represents how much your battery is charged at the moment, compared to its total capacity.
4. Input Depth of Discharge Limit:
Navigate to the field labeled “Depth of Discharge Limit (%)”. Enter the depth of discharge limit as a percentage (%). This value indicates the percentage of the battery that can be used safely without damaging it.
5. Selecting Inverter Usage:
Identify the dropdown menu labeled “Using Inverter”. Choose “Yes” if you are using an inverter with your battery system, otherwise select “No”.
6. Input Inverter Efficiency (If Applicable):
If you selected “Yes” for using an inverter, an additional input field will appear labeled “Inverter Efficiency (%)”. Enter the efficiency of your inverter as a percentage (%). This value reflects how effectively the inverter converts battery power to usable AC power.
7. Input Total Output Load:
Find the field labeled “Total Output Load (W)”. Enter the total power load (in watts) that will be drawn from the battery. This includes all devices or systems that the battery will power.
Performing the Calculation
Calculate Battery Runtime:
After all inputs are filled in, click the button labeled “Calculate Battery Runtime”. The calculator will process your inputs and display the estimated battery runtime in hours under the section “Runtime”.
Handling Errors
Error Checking:
If you see an error message displayed, check to ensure all fields are filled correctly. The error message will guide you to correct any input mistakes.
Resetting the Calculator
Reset for a New Calculation:
To perform a new calculation, click the “Reset” button. This will clear all inputs and reset the calculator for fresh data entry.
General Tips
- Accuracy of Data: For best results, ensure the accuracy of the data you enter, particularly the battery specifications.
- Understanding Limits: The depth of discharge limit is crucial for battery longevity. Avoid setting it too high for lead-acid batteries.
- Inverter Efficiency: Typical inverter efficiencies range from 85% to 95%. If unsure, consult your inverter’s documentation.
The battery runtime calculator is a helpful tool for estimating how long your battery will last under specific conditions. By carefully inputting the correct values and understanding the significance of each parameter, you can effectively plan and manage your battery usage.
Further Examples for Battery Runtime Calculator
Certainly! Let’s go through a few examples of calculations using the battery runtime calculator. I will present these examples in a table format for clarity.
Table with Example Calculations of Various Battery Runtimes
Example | Battery Capacity (Ah) | Battery Voltage (V) | State of Charge (%) | Depth of Discharge (%) | Using Inverter | Inverter Efficiency (%) | Total Output Load (W) | Calculated Runtime (Hours) |
---|---|---|---|---|---|---|---|---|
1 | 100 | 12 | 100 | 80 | No | N/A | 500 | 1.92 |
2 | 200 | 24 | 50 | 50 | Yes | 90 | 1000 | 2.16 |
3 | 150 | 48 | 75 | 60 | Yes | 85 | 750 | 1.44 |
Explanation of Examples
Example 1:
- Battery Capacity: 100 Ah (ampere-hours)
- Battery Voltage: 12 V (volts)
- State of Charge: 100% (fully charged)
- Depth of Discharge: 80% (can use up to 80% of the battery’s capacity)
- Inverter: Not used
- Total Output Load: 500 W (watts)
- Runtime Calculation:
- Since the inverter is not used, the formula simplifies to:
- [ \text{Runtime} = \frac{100 \times 12 \times 1.0 \times 0.8}{500} = 1.92 \text{ hours} ]
Example 2:
- Battery Capacity: 200 Ah
- Battery Voltage: 24 V
- State of Charge: 50% (half charged)
- Depth of Discharge: 50%
- Using Inverter: Yes
- Inverter Efficiency: 90%
- Total Output Load: 1000 W
- Runtime Calculation:
- Considering the inverter’s efficiency:
- [ \text{Runtime} = \frac{200 \times 24 \times 0.5 \times 0.5}{1000 / 0.9} = 2.16 \text{ hours} ]
Example 3:
- Battery Capacity: 150 Ah
- Battery Voltage: 48 V
- State of Charge: 75%
- Depth of Discharge: 60%
- Using Inverter: Yes
- Inverter Efficiency: 85%
- Total Output Load: 750 W
- Runtime Calculation:
- [ \text{Runtime} = \frac{150 \times 48 \times 0.75 \times 0.6}{750 / 0.85} = 1.44 \text{ hours} ]
Key Takeaways for Battery Runtime Calculator
- The runtime decreases as the total output load increases.
- Using an inverter reduces the effective runtime due to efficiency loss.
- Higher battery capacity and voltage lead to longer runtime, but this also depends on the state of charge and depth of discharge.
These examples demonstrate how different factors like battery capacity, voltage, state of charge, depth of discharge, inverter usage, and output load can influence the calculated runtime of a battery.
Visual Chart with Example Battery Runtimes
Here’s a bar chart illustrating the battery runtimes for the three examples we discussed above:
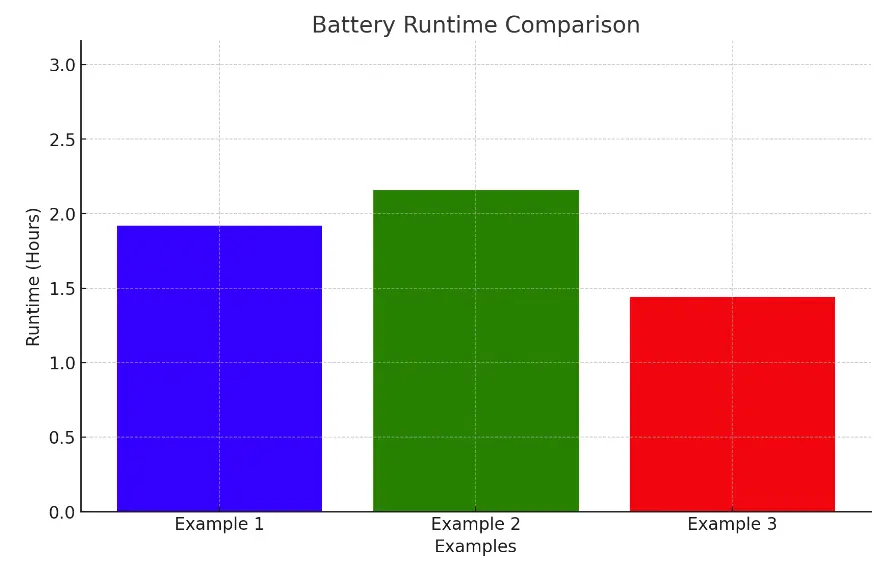
- Example 1 has a runtime of 1.92 hours.
- Example 2 shows a slightly longer runtime of 2.16 hours.
- Example 3 has a runtime of 1.44 hours.
This visual representation makes it easier to compare the different battery runtimes under varying conditions. As you can see, the runtime varies depending on factors like battery capacity, voltage, state of charge, depth of discharge, inverter usage, and output load.
How to Determine How Long a Battery Will Last?
To determine how long a battery will last, we need to understand a few key concepts: battery voltage (measured in volts, V), battery capacity (measured in ampere-hours, Ah), and the power consumption of the device or load the battery is powering (measured in watts, W or amperes, A). The battery’s lifespan depends on its capacity and the load’s power consumption.
Key Concepts:
- Battery Voltage (V): Indicates the electric potential the battery can provide. Common voltages are 12V, 24V, 48V, etc.
- Battery Capacity (Ah): Represents how much charge the battery can hold. A battery with a capacity of 100Ah can theoretically supply 100A for 1 hour, or 1A for 100 hours, under ideal conditions.
- Power Consumption of Load: The amount of power your device or appliance consumes. It’s often measured in watts (W) or amperes (A).
- Battery Life Calculation: Battery life is generally calculated using the formula: Battery Life = Battery Capacity (Ah) / Load Current (A). This formula assumes the voltage of the battery matches the voltage requirement of the load.
Battery Life Examples:
- 12V Battery Life:
- Assuming a 12V battery with a certain Ah rating, the life will depend on the current drawn. For a 12V, 100Ah battery supplying a 10A load, the battery life would be approximately 10 hours.
- 24V Battery Life:
- A 24V battery’s life also depends on its Ah rating and the load. If we have a 24V, 200Ah battery powering a 20A device, it would last around 10 hours.
- 48V Battery Life:
- For a 48V system, the same principle applies. A 48V, 300Ah battery powering a 30A appliance would last for about 10 hours.
The calculations for specific Ah ratings like 70Ah, 110Ah, 300Ah, 600Ah, 150Ah, and 200Ah follow the same principle. However, it’s essential to know the load’s power consumption to provide accurate estimates.
Tables with Examples:
Example 1: Battery Life for Different Ah Ratings (Assuming 10A Load)
Battery Capacity (Ah) | Battery Life (Hours) @ 10A Load |
---|---|
70Ah | 7 hours |
110Ah | 11 hours |
300Ah | 30 hours |
600Ah | 60 hours |
150Ah | 15 hours |
200Ah | 20 hours |
Example 2: Battery Life for 12V, 24V, 48V Batteries (Assuming 100Ah Capacity, 10A Load)
Battery Voltage | Battery Capacity (Ah) | Load (A) | Battery Life (Hours) |
---|---|---|---|
12V | 100Ah | 10A | 10 hours |
24V | 100Ah | 10A | 10 hours |
48V | 100Ah | 10A | 10 hours |
Considerations:
- These examples assume ideal conditions. In reality, battery efficiency, temperature, age, and discharge rate can affect battery life.
- For devices that require watts, conversion from amperes to watts is necessary, using the formula: Power (W) = Voltage (V) × Current (A).
- Deep cycle batteries are designed for sustained discharge and may have different characteristics compared to standard batteries.
In conclusion, the duration a battery will last depends significantly on its capacity and the power consumption of the load it’s powering. The above tables provide a basic guideline under ideal conditions. For more precise estimations, specific details about the load and battery type are needed.
Different Ways on How to Calculate Your Battery Runtime
Calculating battery runtime is a valuable skill, especially when dealing with electronics, emergency power supplies, or even planning for outdoor activities where battery power is essential. There are two primary methods to calculate battery runtime: the Basic Method and the Peukert’s Law Method.
1. Basic Method (Simple Ampere-Hour Calculation)
The Basic Method is straightforward and is based on the battery’s ampere-hour (Ah) rating, which indicates how much current a battery can supply over a specific period. Here’s how to do it:
Instructions:
- Identify Battery Capacity: Determine the battery’s capacity in ampere-hours (Ah). This information is typically provided by the manufacturer and can be found on the battery label.
- Determine Load Current: Find out the current (in amperes, A) that your device draws from the battery. This can usually be found in the device’s specifications or calculated by dividing the power (in watts, W) by the voltage (in volts, V).

Considerations:
- This method assumes ideal conditions and may not account for real-world factors like battery aging, temperature, and efficiency losses.
- The load should be consistent for accurate results.
2. Peukert’s Law Method (Advanced Calculation)
Peukert’s Law provides a more accurate estimation, especially for lead-acid batteries. It takes into account the fact that higher loads reduce the effective capacity of a battery.
Instructions:
- Identify Battery Capacity and Peukert Number: Find the battery’s rated capacity (Ah) and its Peukert number. The Peukert number is a constant specific to each battery, often provided by the manufacturer.
- Determine Load Current: As with the basic method, find out the current drawn by your device.
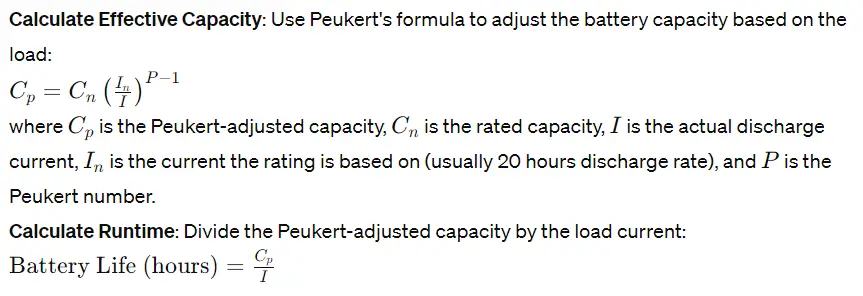
Considerations:
- This method is more complex but gives a more accurate estimation for lead-acid batteries under higher loads.
- The Peukert number is crucial for accuracy and might be hard to find for some batteries.
- This method is generally not necessary for lithium-ion batteries, as they have a more linear discharge rate.
Both methods provide a fundamental understanding of how long a battery will last under specific conditions. The Basic Method is suitable for quick, rough estimates, while Peukert’s Law Method is better for precise calculations, especially in high-load or critical applications. Remember, these methods provide estimates and actual performance can vary based on numerous factors including battery type, age, and temperature.
FAQ Section
Q1: What is the importance of knowing a battery’s runtime? A: Knowing a battery’s runtime helps in planning and ensures that your battery can meet the power demands of your devices without running out unexpectedly.
Q2: Why is the Depth of Discharge important? A: The DoD limit is crucial as discharging a battery beyond its recommended limit can significantly reduce its lifespan and efficiency.
Q3: Can I use this calculator for any battery type? A: Yes, this calculator is versatile and can be used for various battery types, including lead-acid and lithium batteries.
Q4: How accurate is the battery runtime calculation? A: While the calculation provides a good estimate, actual runtime can vary due to factors like battery age, temperature, and the efficiency of connected devices.
Q5: Does higher capacity always mean longer runtime? A: Not necessarily. Runtime also depends on the load and how efficiently the battery discharges its stored energy.
Glossary of Battery Terms
- Ampere (A): A unit of electric current. Batteries supply current to devices, measured in amps.
Think of it like water flowing through a pipe – more amps mean more flow. - Ampere-Hour (Ah): A unit measuring battery capacity. A 100Ah battery can supply 1 amp for 100 hours or 10 amps for 10 hours.
It tells you how long a battery can deliver power. - Battery Management System (BMS): An electronic system that manages and protects batteries from overcharging, deep discharging, and overheating.
Your battery’s ‘brain’ ensuring safe operation. - Capacity: The amount of charge a battery can store, typically measured in Ah or Wh.
Higher capacity means longer runtime. - Cycle Life: The number of complete charge and discharge cycles a battery can undergo before its capacity drops significantly.
More cycles mean a longer-lasting battery. - Depth of Discharge (DoD): The percentage of a battery’s capacity used before recharging.
A 50% DoD means using half the battery’s capacity before recharging. - Energy Density: The amount of energy stored per unit weight or volume, measured in Wh/kg.
Higher density means more power in a smaller, lighter battery. - Inverter: A device that converts DC power from batteries to AC power for household appliances.
It lets you use battery power for standard electronics. - Inverter Efficiency: The percentage of battery power that gets converted to usable AC power.
A 90% efficiency means 10% is lost as heat. - Lithium-Ion Battery: A rechargeable battery known for high energy density, long cycle life, and lightweight.
Common in laptops, EVs, and solar storage. - Peukert’s Law: A formula that shows how battery capacity decreases as the discharge current increases, especially in lead-acid batteries.
Higher loads drain your battery faster than expected. - Parallel Connection: Connecting batteries side by side to increase capacity while keeping the voltage the same.
More capacity, same voltage. - Series Connection: Connecting batteries end-to-end to increase voltage while keeping the capacity the same.
Higher voltage, same capacity. - State of Charge (SoC): The current charge level of a battery as a percentage of its full capacity.
100% SoC means fully charged. - Watt (W): A unit of power measuring how fast energy is used.
More watts mean more power consumption.
- Watt-Hour (Wh): A unit measuring energy consumption over time.
A 100W device running for 10 hours uses 1000Wh.
Additional Online Reading Materials for Battery Runtime Calculator
We found several informative online sources that can help you understand more about battery life and factors affecting it. Here’s a summary of their key points:
AAA Automotive:
- Car batteries typically last between 3 to 5 years. The lifespan can be influenced by factors such as time, heat, and vibration.
- Batteries in hotter climates may degrade faster, while those in cooler climates can last longer.
- Maintenance chargers can extend a car battery’s service life, especially for vehicles that are driven infrequently or parked for extended periods.
- A malfunctioning charging system can also reduce battery life.
- Regular battery inspections and testing, especially after the third year, can help identify potential issues early.
- AAA Automotive
AutoZone:
- Factors affecting car battery life include slow discharging/recharging, structural failure, and issues with the alternator.
- Signs of a failing battery can be slow starting, leakage or corrosion, sudden failure to start, and bad smells indicating sulfuric acid.
- To prevent battery failure, it’s recommended to use a battery maintainer if the vehicle isn’t used often, keep the battery terminals clean, avoid using the battery for powering accessories for extended periods, and test the battery frequently.
- AutoZone
DigiKey Electronics – Battery Life Calculator:
- This tool estimates battery life based on the nominal battery capacity and the average current drawn by a device.
- Battery capacity is typically measured in Amp-hours (Ah) or milliamp-hours (mAh), with Watt-hours (Wh) occasionally used.
- Factors such as battery condition, age, temperature, and discharge rate can affect actual battery life.
- DigiKey Electronics
These sources provide a comprehensive understanding of how various factors impact battery life and offer practical tips on maintaining and extending the lifespan of batteries. They are particularly useful for car batteries, but the principles can be applied broadly to other types of batteries as well.